Biomechanics and Bioengineering of Orthopaedics: Biomaterials as biologic facilitators
Over centuries we can appreciate the advancement in biomaterials; beginning with a basic linen thread for wound healing, metallic sutures with golden wires and metals for hard tissue replacements we then came to the age of using bioinert materials for implants and currently we are witnessing the era of biomimetic materials, hydrogels and 3D bioprinting (Fig. 9-1).
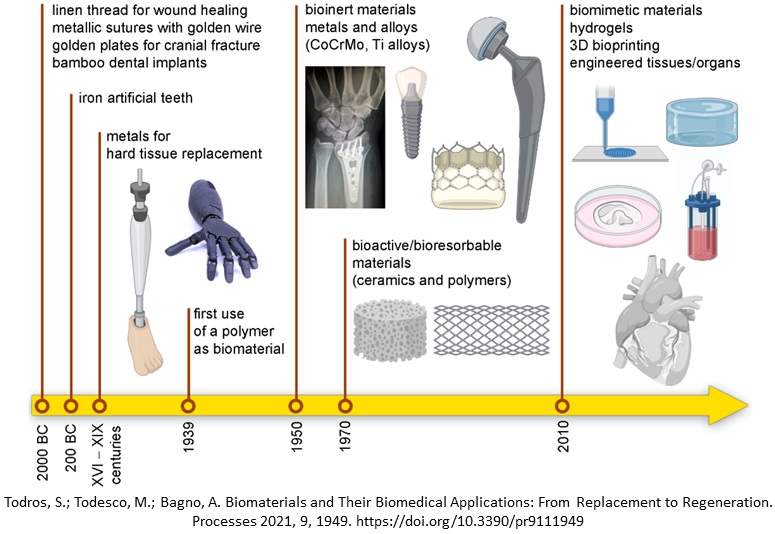
Properties of biomaterials
When dealing with biomaterials we consider the biological, mechanical, physical and chemical. Out of these properties, the most important to carefully look at is the biological properties. That is, are the materials bioactive, bioinert or biodegradable in vitro or in vivo.
That’s where biocompatibility comes in; it’s a general term for how compatible a material is with living tissue. It is not only an important feature of biomaterials, it is what makes something a biomaterial. There are several ways a material can interact with your body; it can hurt you, it can dissolve and be replaced by cells, your body can surround it with a protective layer or it can bond with your living tissue. Biocompatible materials should result in a healthy response. They don’t tend to cause blood clots or infections and lead to normal, uncomplicate healing. Metals, ceramics, polymers, composites, living cells and tissues can all be candidates for biomaterials as long as they have the right skills for the job.
Metals
Metals are composed of atoms joined by bonds. Now taking a look at 3 key metals often used in biomaterial applications. Stainless steel 3, 16, L. This means in terms of composition it has 3% molybdenum; 16% nickel and the L stands for low carbon. Next is titanium, TI64V and that is because it has a composition of 6% aluminium; 4% vanadium and 90% titanium. Finally cobalt chromium; composition is 60% cobalt, 25% chromium and 5% molybdenum (Fig. 9-2).
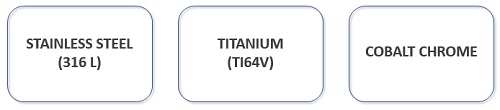
Common metals used as biomaterials are stainless steel, cobalt chromium alloys and titanium as mentioned earlier. The table in Fig. 9-3 shows some of their mechanical properties such as young’s modulus, yield strength and tensile strength, compared to cortical bone.
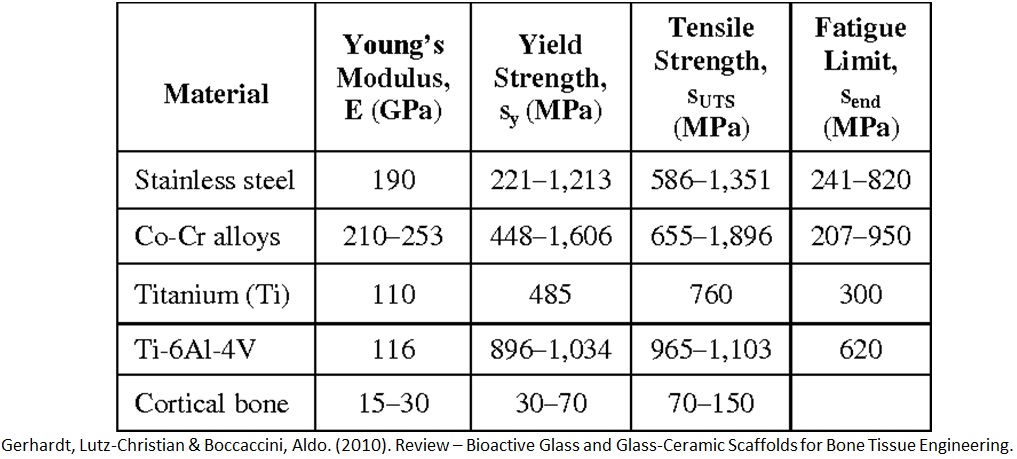
Titanium and stainless steel are used everywhere from joint replacements to dental implants (Fig. 9-4). They are biologically inert materials which means they cause little or no reaction with nearby tissues. The body may recognize them as foreign materials and try to surround them in fibrous tissue but would not outright reject them. Titanium is however often used in place of stainless steel as it lacks compounds like nickel has been found to be toxic and may cause allergic reactions. Titanium also has a lower density compared to other metallic biomaterials, so a titanium implant will be lighter than a similar one made of stainless steel. Both titanium and stainless steel have good mechanical properties: strength, resistance to bending and durability, making them excellent candidates for things that tend to get a lot of wear and tear like joints. Titanium is also used for coating non-biocompatible materials.
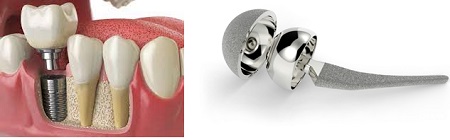
Ceramics
A ceramic is a composite material made of metallic and non-metallic elements joined by covalent bonds (Fig. 9-5). It’s got 2 types; a bioinert type such as alumina or a bioactive form such as hydroxyapatite. For its properties, ceramics are brittle which means it’s got a very little plastic zone before failure, wettable means it’s got a high affinity for lubricants, hard means it’s resistant to scratching, inert means it’s not reactive chemically and efficient is it’s got a low coefficient of friction. In terms of its uses, it’s used as a bearing surface.
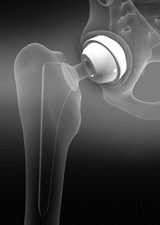
Polyurethane
Sometimes we may need more flexible biomaterials and for that engineers often turn to polyurethane, a type of polymer used in catheters, artificial heart valves and other flexible devices and hydrogels. It has a high level of elasticity, so is pretty flexible, but it’s also durable and resistant to tearing making it perform well under both static and dynamic loading conditions. This polymer is blood-friendly because platelet cells which cause clotting do not stick to them as much (Fig. 9-6).
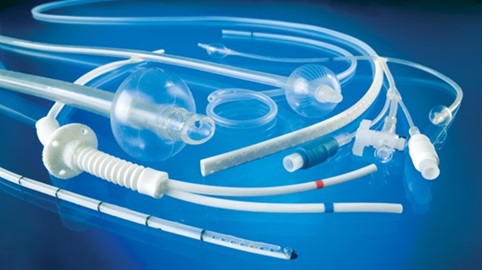
Hydrogels
Hydrogels are materials made up of things called hydrophilic structures, crosslinked polymers which are capable of holding large amounts of water. For example, one of the most widely used hydrogels is Phema (Fig. 9-7), a material that is pretty unreactive and supportive of biological processes. It’s tough, doesn’t degrade easily, isn’t absorbed by the body and it can take on many shapes and forms. Their unique properties make them perfect candidates for drug delivery.
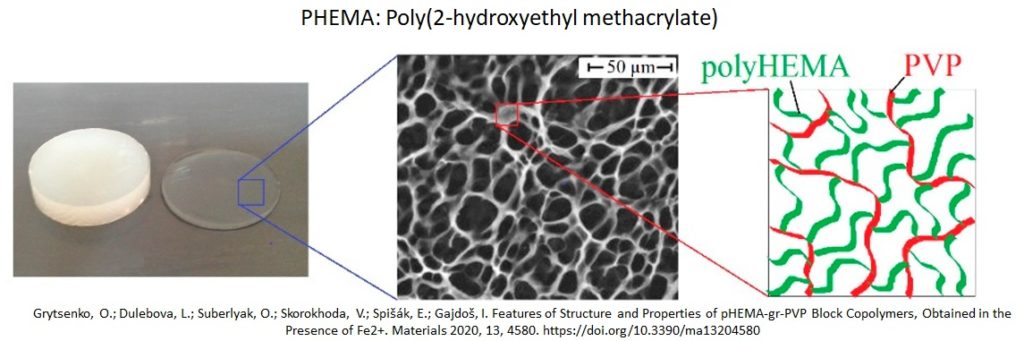
Nanoparticles
Nanoparticles are materials with overall dimensions in the nanoscale, that is, under 100 nanometers. For comparison, cells in living organisms are typically 10 micrometers across, literally giants next to nanoparticles (Fig. 9-8). Proteins measure about 5 nanometers, which is about the scale of the smallest nanoparticles.
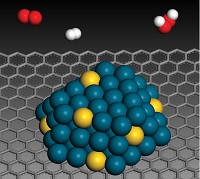
They are undetectable by the human eye and can exhibit significantly different physical and chemical properties compared to their larger material counterparts, that is their material properties change as their size approaches the atomic scale. For example, copper is considered a soft material, with bulk copper bending when its atoms cluster at the 50 nanometer scale. Consequently, copper nanoparticles smaller than 50 nanometer are considered a very hard material, with drastically different malleability and ductility performance when compared to bulk copper.
Nanomaterials can occur naturally, be created as the by-products of combustion reactions, or be produced purposefully through engineering to perform a specialized function. Nano materials can be composed of metals, ceramics, polymers, organic materials and composites. Applications of nanoparticles are as follows.
Biosensors: Nanowires, nanotubes, quantum dots, and other nanoparticles are contributing to the next generation of biosensors geared towards improving the diagnosis of disease. Implantable biosensors are devices that can evaluate signs and symptoms of disease and regulate drug release, such as insulin for diabetics.
Drug delivery systems: Nanoparticles are engineered with an attraction to diseased cells, allowing treatment to be delivered directly to damaged cells and reducing damage to healthy cells. Nanoparticles can be engineered to precisely deliver drugs, light, and heat to specific cells in the body to treat cancer and other illnesses.
Tissue engineering: Nanotechnology used in tissue engineering helps repair, reproduce, or reshape damaged tissues. Successful nanotechnology tissue engineering could replace organ transplants and artificial implants; graphene and other particles are already being tested in advanced bone tissue engineering applications.
Nanomaterials are contributing significantly to tissue engineering advancements for bone, cartilage, vascular, neural and bladder applications. For example, an injectable hydrogel can be mixed with nanoparticle components which contain molecules which are signals for cells to proliferate and differentiate into specialized osteoblast cells (Fig. 9-9). This technology is used for regeneration of bone in fractures and osteoporosis disease.
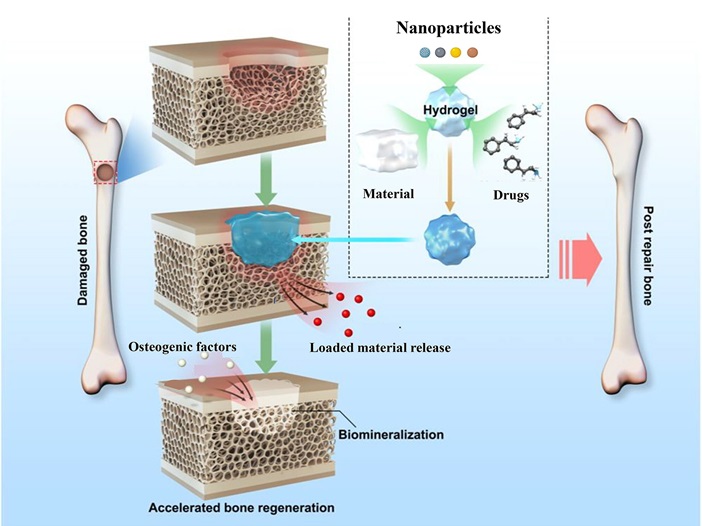